Research PR showcase! Vol.5: Novel agriculture rooted in imaging biology – work with the sun (endogenous mechanism) instead of the north wind (forceful manipulation)
IRCAEB Dept. of Basic Research, Takumi Higaki
Research PR Showcase Vol.5 will feature the works of Prof. Takumi Higaki, a researcher in the brand-new field of imaging biology. Prof. Higaki specializes in analyzing how cells divide and how proteins move within cells, as well as conducting simulations of these events. One may be surprised
that math-heavy activities such as programming and computational simulations
are routine works for “imaging” analysis, but it nevertheless takes a bit
of artistic sense to present these findings in a visually-pleasant way.
The main subject of the research introduced here will be the Arabidopsis
stomata (singular: stoma). Arabidopsis are cruciferous plants with leaves near the ground and stems that grow
from the plant’s center, bearing small white flowers. Since the late 1980s
Arabidopsis has been used as a model plant species in laboratories around the world.
Similar to animals, plants convert oxygen and glucose into carbon dioxide
(CO2) and energy (ATP) during respiration. Meanwhile, in the presence of light plants can also convert CO2 and water
into glucose (used both an energy source and structural components in cells)
through photosynthesis. With the urgent needs in greenhouse gas reduction, photosynthesis has
gained much attention due to its ability to consume the greenhouse gas
CO2.
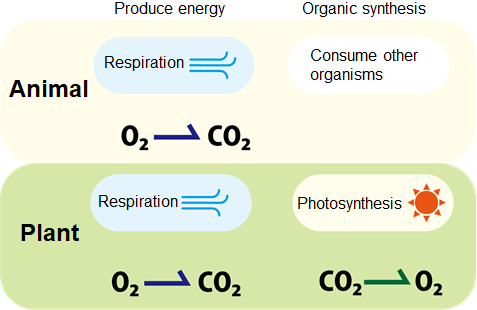
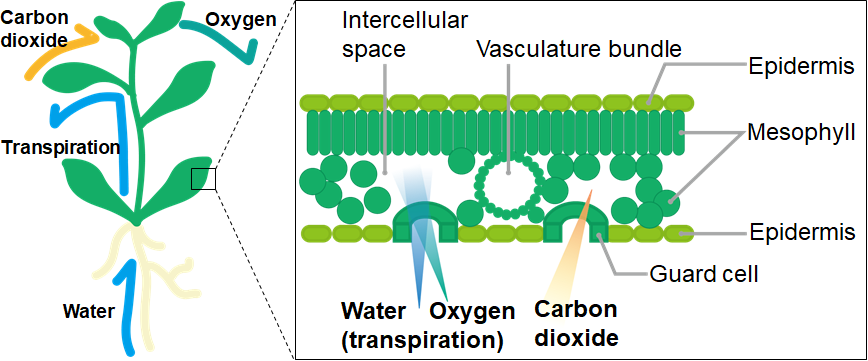
So where do plants take in and expel CO2 and oxygen? Many animals conduct gas exchange through orifices like the nostrils or the mouth, or even directly through the skin. Plants do not have the equivalents of nostrils nor mouths, but do possess mouth-like microscopic structures called stomata on leaf surfaces. 1 mm2 of Arabidopsis leaf can contain up to 100 stomata! The lip-like guard cells open the stomata, which take in CO2 while expelling water (vapor) and oxygen.
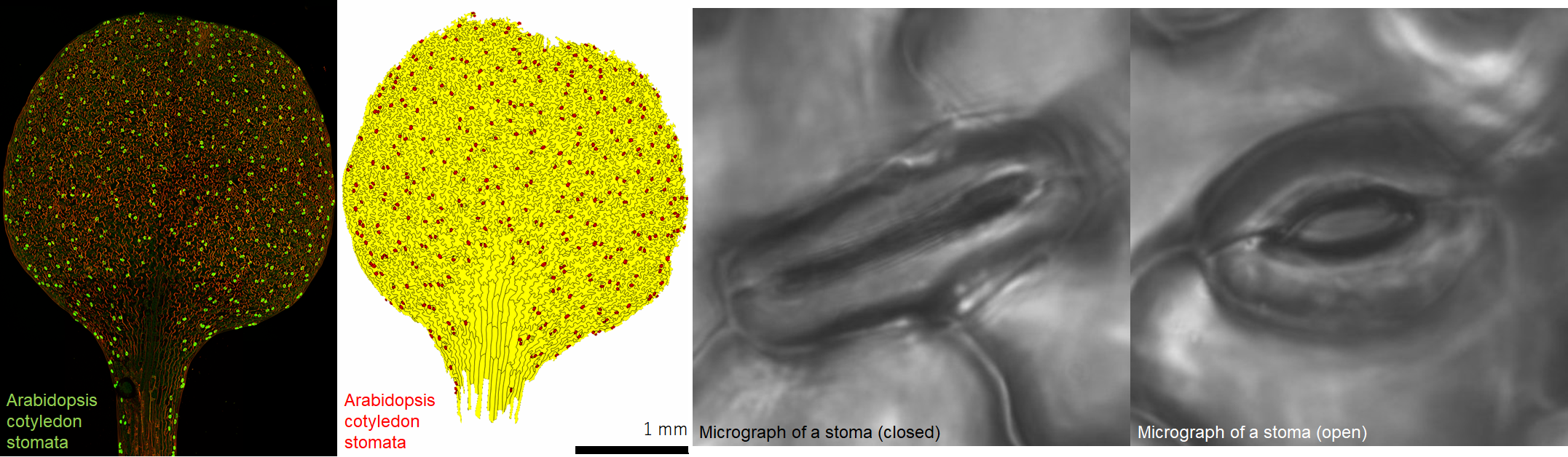
The shapes of the guard cells dictate whether a stoma opens or closes. The cell walls on the inner sides of guard cells (where two guard cells meet when a stoma closes) tend to be thick, while the cell walls of the outer regions are thinner. This way, when a guard cell expands it curves outward, which opens the stoma. The reverse happens when a guard cell shrinks and straightens, which closes the stoma. Guard cells change their sizes by adjusting the cellular osmotic pressure, which in turn determines whether water flows in or out of the cells. Stomata closure is tightly regulated by various environmental cues including light, water and CO2 levels. By artificially manipulating stomata closure, it may be possible to enhance CO2 uptake and thereby improve photosynthesis efficiencies of plants.

So what are the mechanisms that converts environmental cues into guard cell osmotic pressure changes? One of the environmental cues known to open stomata, blue light, is perceived by guard cell surface proteins called phototropin. Once light is perceived, phototropin activates proton pumps on guard cell surfaces, which eject protons out of guard cells. This builds electrical potential differences across the guard cell membrane, which causes ions K+, Cl- and water to flow into guard cell, making the guard cell expand. When stomata close the stored K+ ions are pumped out of guard cells, causing water to flow out of guard cells.
Since proton pumps directly regulate the osmotic pressures in guard cells, their localizationon guard cell surfaces are exceptionally important. Complex regulatory mechanisms are indeed involved in the transport of proton pumps to the cell surface. In 2013, using an Arabidopsis mutant that opens its stomata constitutively even in low CO2, the PATROL1gene was identified as a regulator of proton pump localization. In the patrol1 mutant, proton pumps that should only be present on guard cell surfaces were also found within guard cell cytoplasm, suggesting PATROL1 is required for proper proton pump localization.
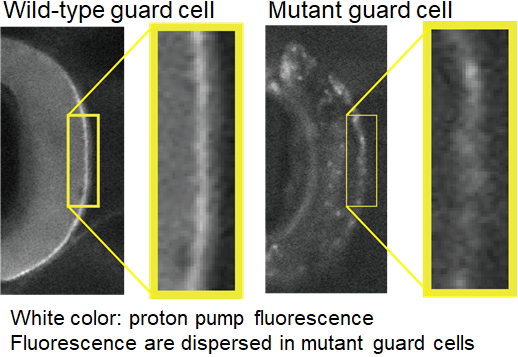
PATROL1 is structurally related to animal proteins that regulate neurotransmitter secretion. Specifically, it resembles the Munc13-1 protein that mediate the fusion of vesicles with neuron cell membranes to release neurotransmitters (green particles in the diagram).
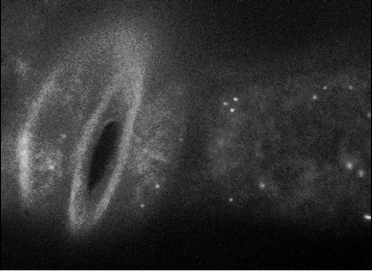
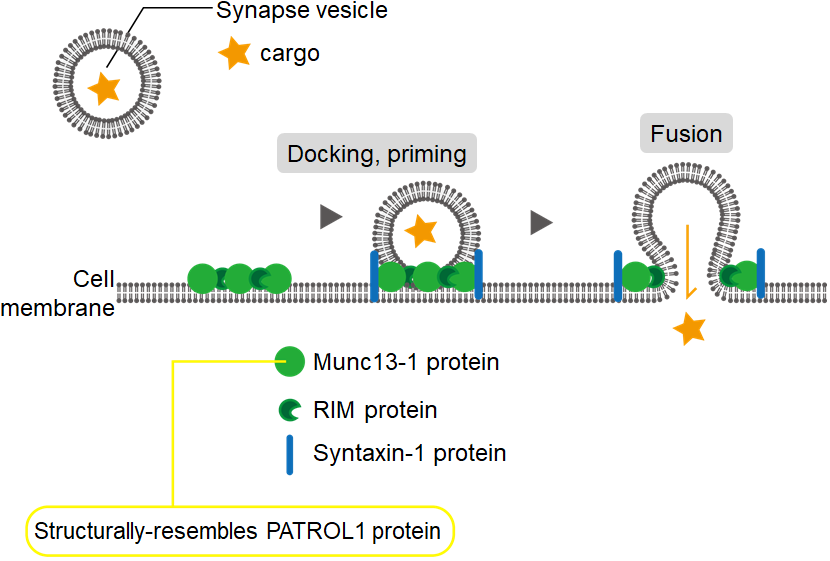
In order to understand how neuronal secretion is related to stomata opening,
we observed PATROL1 proteins in guard cells using live-imaging. Expectedly,
vesicle fusions similar to those seen in neurons were also observed in
guard cells, suggesting proton pumps may be translocated to guard cell
surfaces through vesicle fusion with the cell membrane.
Similarly, over-expressing PATROL1 causes plants to grow larger. Assuming guard cell proton pumps are delivered more efficiently to the
cell membrane, photosynthesis efficiency may have increased. and plants are then able to grow larger as a result.
Thus theoretically with more proton pumps on guard cell surfaces, crop plants should be able absorb more CO2 and increase crop yield, even with light intensity unchanged. As such, the viability of this approach to improve agricultural production has been considered. However, due to the lack of understanding of other factors that regulate guard cell proton pump localization, and how they respond to complex environmental factors, manipulating PATROL1 alone may cause unintended consequences.
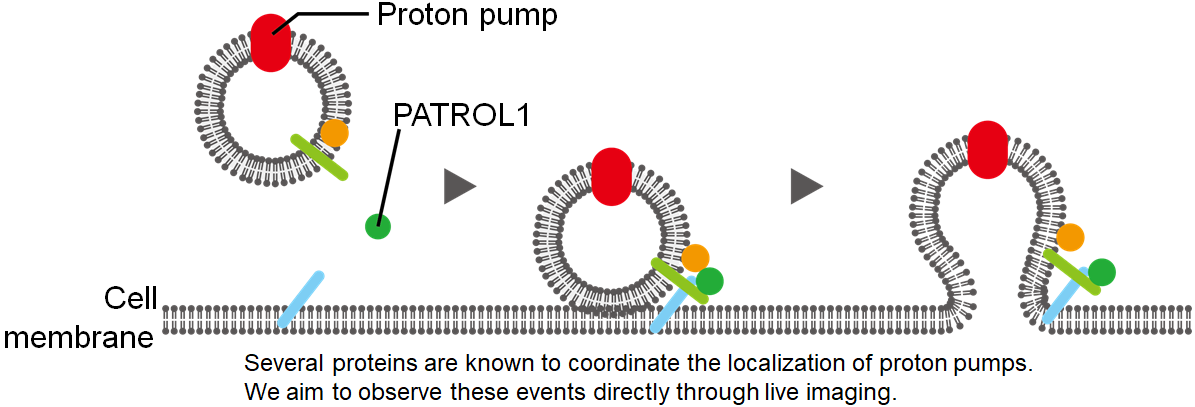
To address this problem, we plan to first observe guard cell proton pump localization under different environmental conditions using live-imaging (observing the proton pumps in real-time while the plant is alive). Live-imaging isn’t just about taking pictures – the hard part comes when these pictures are quantified and analyzed. Live-imaging workflow can be divided into 3 phases: Image acquisition, processing and evaluation. Factors in all 3 phases can significantly affect the conclusion of the overall image analysis. For example, during image acquisition plants must be exposed to laser to be imaged (GFP fusion proteins fluoresce when shone by light of a specific wave length), however too much laser exposure is detrimental to plant growth and should be avoided. Next during image processing, various parametersmust be manually defined (e.g. when should an image be deemed not useful to be discarded). When the imaging subject and/or conditions are changed, these parameters must be re-evaluated accordingly, making image processing the bottleneckduring live-imaging analysis. However, with the implementation of AIwe foresee the efficiency of live-imaging workflow to be improved dramatically in the future.

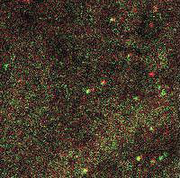
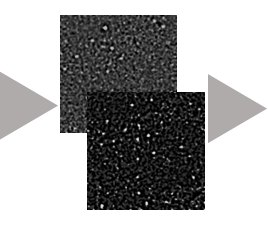
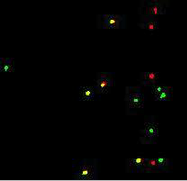
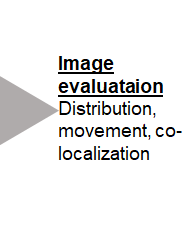
Our agricultural research from here on will focus not only on how proton pump activities regulate stomata opening, but more on using pre-existing regulatory mechanisms in plants to enhance growth, such as accelerate the proton pump localization responses to environmental cues. Like Aesop’s fable “The North Wind and the Sun”, we hope to develop strategies for plants to more efficiently fix CO2 even under unfavorable conditions by working with, not against, the plant’s endogenous regulatory mechanisms. Using imaging biology to analyze large numbers of images, it may eventually be possible to re-construct the special and temporal aspects of many proteins and their functions in cells. By enhancing the responses of these processes, we may be able to conjure novel concepts to improve agricultural productions while minimizing impacts on the environment.